Delving into the skin layers
The human skin, our body’s largest organ, is more than just a protective barrier. It is a complex organ comprising various layers with distinct structures and functions (Figure 1). The epidermis consists of keratinocytes, melanocytes, and Langerhans cells, while the dermis contains fibroblasts, blood vessels, and extracellular matrix components.1 The hypodermis primarily comprises adipocytes and connective tissues, contributing to insulation and cushioning.
Recreating this intricate structure in the lab has been a major challenge for researchers, but 3D skin models are bringing us closer than ever to mimicking human skin. In this article, we explore the unique characteristics of 3D skin models, delving into their advantages over traditional 2D cultures, their applications in dermatology research, challenges, and the promising future they hold.
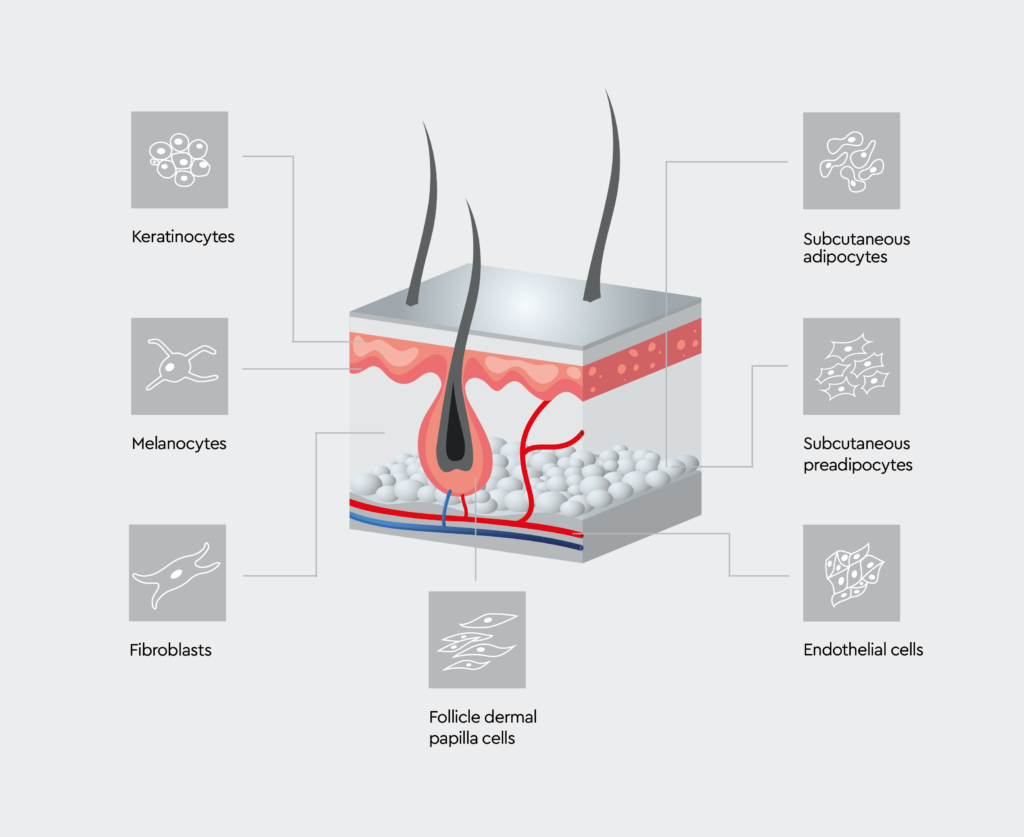
Importance of in vitro models in skin research
Understanding the intricacies of the human skin is crucial for advancements in dermatology. In vitro models provide a controlled environment for studying the complex interactions within the skin. Before the development of 3D skin models, dermatological studies primarily relied on 2D cultures of human skin cells and animal models.2 While these models provided valuable insights into certain aspects of human skin biology, their limitations, such as the inability to mimic the native skin environment accurately, caused efforts to establish more physiologically relevant alternatives that do not rely on animal testing.3
Advantages of a 3D human skin model
Although valuable, traditional 2D cultures lack the complexity of 3D models and fail to capture the intricacies of cell interactions and 3D architecture found in natural skin. The use of a 3D model of skin offers several advantages:
- Resemblance to natural skin composition: 3D cultures accurately mimic the composition and architecture of the human skin, providing a more realistic model.4
- Skin complexity: The inclusion of multiple cell types, growth factors, and extracellular matrix (ECM) components contributes to the recreation of skin complexity in 3D models.5
- More realistic environment: They provide a more realistic cellular environment that closely mimics the natural structure and function of the human skin.6 This allows for more accurate studies on skin biology and pathology.
- Improved physiological relevance: The three-dimensional nature of 3D skin models allows for better representation of cell-cell and cell-matrix interactions, which are crucial for understanding the mechanisms of skin diseases and conditions.7
- Enhanced predictability and reproducibility: They offer a more reliable and reproducible platform for testing various compounds, ensuring that results obtained are more predictive of in vivo responses.8
- Possibility of drug penetration studies: 3D skin models are excellent tools for studying the penetration and efficacy of topical drugs and cosmetics.8 They can provide valuable insights into how these substances interact with the skin and their potential effects, offering a more ethical alternative to animal testing for pharmacological and skincare studies.
These advantages make 3D skin models a powerful tool in dermatology research and hold great promise for personalized medicine and drug discovery.
Types of 3D skin models
As bioengineering technologies evolve, various approaches have been developed to construct 3D skin models. Each type of 3D skin model offers unique insights into different aspects of skin biology and disease, and human skin models can be customized based on specific research needs.
- Full-thickness skin models incorporate both the epidermal and dermal layers of the skin, providing a comprehensive platform for studying skin biology.9 They are particularly useful for investigating wound healing and the effects of aging on the skin.
- Epidermal models focus solely on the epidermis, the outermost layer of the skin. They are commonly used for studying skin barrier functions and the effects of UV radiation.10
- Melanoma models incorporate melanocytes, which are responsible for melanin production. They are crucial for studying melanoma, the most dangerous type of skin cancer.11
- Hair follicle models include hair follicles, providing a unique platform for studying hair growth and disorders such as alopecia.12
- Vascularized skin models incorporate blood vessels, allowing for the study of angiogenesis and the interaction between skin cells and blood vessels.13
Applications of human 3D skin models
In the ever-evolving landscape of dermatology research, the integration of 3D skin models has ushered in a new era of possibilities and advancements. Because of the remarkable versatility of 3D cultures, the use of 3D models of human skin extends across various fields. From unraveling the mechanisms of skin diseases to pioneering breakthroughs in cosmetic research, 3D skin models have become indispensable tools in various scientific domains, offering a nuanced understanding of human skin physiology (Figure 2).
Applications of 3D models of human skin include the following:
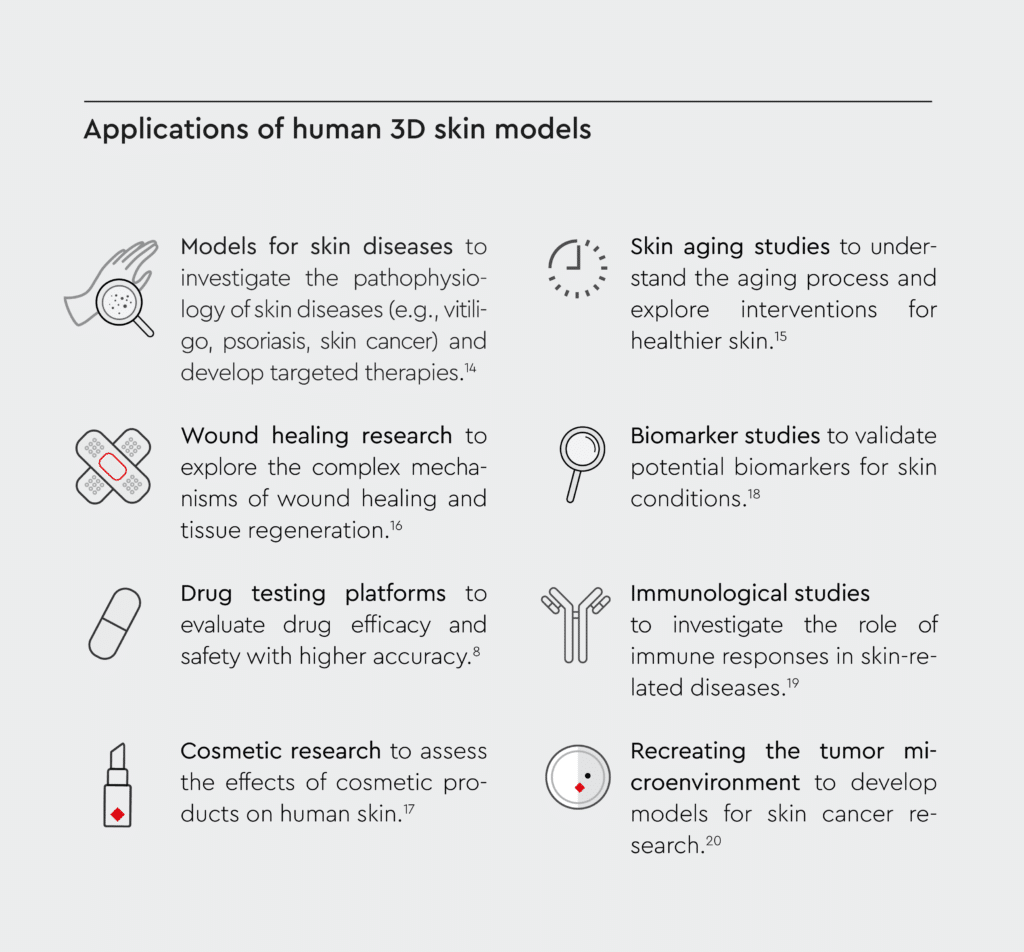
Emerging technologies
Heralding a new era in dermatological research, 3D skin models present several opportunities alongside distinct challenges. Despite their vast potential, these advanced models face obstacles to adoption. Understanding these obstacles is key for the development of new technologies to leverage the full potential of 3D skin models.
Challenges of using 3D skin models
Although 3D skin models offer several advantages over conventional 2D cultures, there are barriers to their widespread adoption.
- Standardization: Achieving consistency across different laboratories and research settings remains a challenge.21 Establishing standardized protocols for the generation of 3D skin models is crucial to ensure the reproducibility and comparability of results.
- Reproducibility and scalability: As research demands grow, the ability to reproduce results reliably and scale up production becomes paramount. Researchers are actively exploring strategies to enhance the reproducibility and scalability of 3D skin models, making them more accessible for a broader range of studies.22 Cell expansion services can also aid in the reproducible and standardized expansion of skin cells that can be used to develop 3D models.
- Complexity: Although 3D skin models are more physiologically relevant than 2D cultures, the complexity of the human skin makes it challenging for in vitro models to fully recapitulate.23
Exploring the full potential of 3D models of skin
The field of 3D skin models is evolving rapidly, with continuous advancements in technology and potential applications (Figure 3). Emerging techniques, such as advanced bioprinting and improved organ-on-a-chip systems, show promise in addressing the current challenges facing the broader adoption of 3D skin models and bridging the gap between in vitro and in vivo studies.
Promising emerging technologies include the following:
- Bioprinted skin: This cutting-edge technology involves the layer-by-layer deposition of cells, biomaterials, and bioactive molecules to create a three-dimensional structure. Bioprinting enables precise control over the spatial distribution of cells, mimicking the complex architecture of human skin.24 This method may provide the answer to vascularization of skin models.
- Scaffold/matrix-based models: Utilizing scaffolds provides a framework for cell attachment and growth, promoting the development of a more structured and organized model that replicates the in vivo architecture of the skin.25
- Organoids: Organoid systems are developed from skin cells and offer the potential to replicate the complex tissue microstructure of the skin, including features such as sweat glands, hair follicles, and sensory neurons.26
- Organ-on-a-chip systems: Mimicking the physiological microenvironment of the skin, organ-on-a-chip systems integrate various cell types and microfluidic channels. These systems offer an advanced platform to study cell interactions, drug responses, and tissue development.27
- Hanging drop methods: This method uses self-assembled spherical clusters of cell colonies to grow the 3D skin model, offering an environment that mimics physiological systems more closely than systems using ECM or hydrogels.28 This simple and reproducible method offers an environment that mimics cell-cell interactions more closely than matrix-based systems.
- Human ex vivo skin explants: Although explants offer physiologically relevant models for drug screening, there is little opportunity to control experimental variables, and obtaining samples from donors can be challenging.9
- Magnetic levitation: This method enables the development of 3D ring constructs and is suitable for high-throughput screening. By controlling the magnetic field, the geometry and clustering of different cell types can be regulated to mimic gene expression, signaling, and morphology under in vivo conditions.29
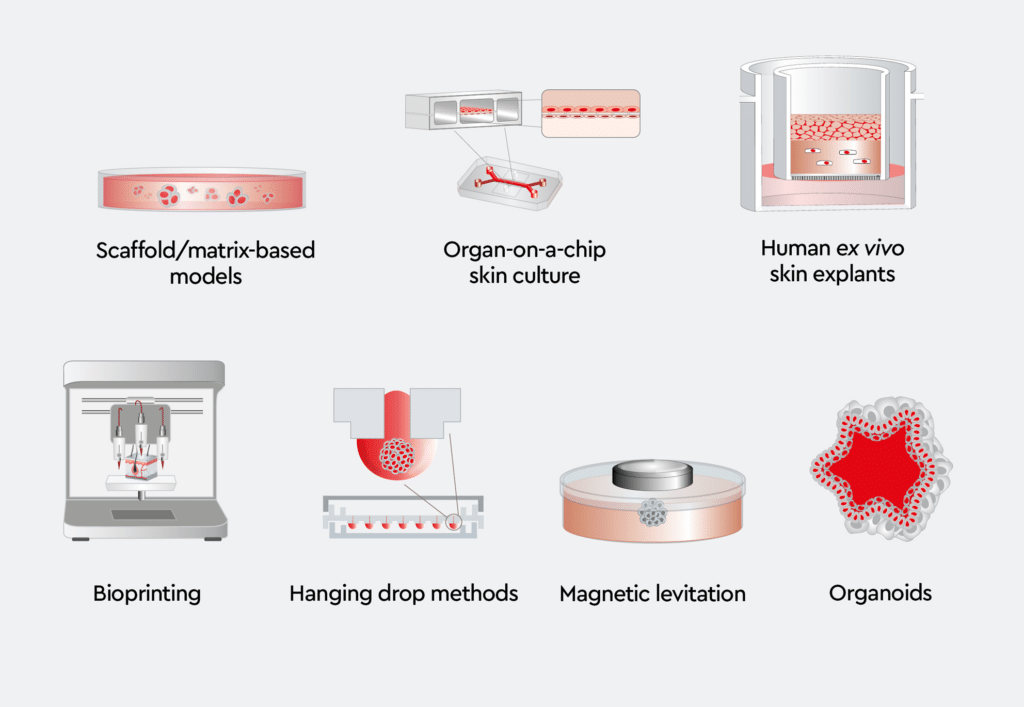
Advances in bioprinting and tissue engineering are paving the way for more sophisticated and realistic 3D skin models. These technologies could allow for the creation of patient-specific 3D skin models based on a patient’s own cells, which can be used for personalized drug testing and could pave the way for personalized treatments in dermatology.
Innovative solutions for 3D skin models
As cell culture experts, we remain at the forefront of innovations in 3D cultures, constantly updating our products to align with the latest technological trends. We provide a comprehensive portfolio of products that help you establish a 3D skin model. These products include:
- Normal Human Epidermal Keratinocytes (NHEK) GM3 are primary cells isolated from the epidermis of human skin. They are crucial for creating a realistic epidermal layer in 3D skin models.
- Normal Human Epidermal Melanocytes M3 (NHEM) M3 produce melanin, the pigment responsible for skin color. They are essential for studying pigmentation disorders and melanoma in 3D skin models.
- Normal Human Dermal Fibroblasts (NHDF) form the dermal layer of the skin and produce ECM. They play a vital role in wound healing and aging studies.
- Human Follicle Dermal Papilla Cells (HFDPC) are found at the base of hair follicles and play a key role in hair growth and cycling. Isolated from the human dermis of the scalp, our HFDPCs are useful for creating 3D skin models that incorporate hair follicles.
- Keratinocyte Growth Medium 3 is a specialized medium designed to support the growth of keratinocytes. It ensures their optimal growth and health for the development of high throughput 3D in vitro wound healing models, among other applications.
- Fibroblast Growth Medium 2 supports the growth of human fibroblasts in 3D in vitro wound healing models.
- Melanocyte Growth Medium M3 is designed to optimize the growth of melanocytes.
- Follicle Dermal Papilla Cell Growth Medium supports the growth of follicle dermal papilla cells.
References
Expand
- Vestita M, Tedeschi P, Bonamonte D. Anatomy and physiology of the skin. In: Maruccia M, Giudice G, eds. Textbook of Plastic and Reconstructive Surgery: Basic Principles and New Perspectives. Springer International Publishing; 2022:3-13. doi:10.1007/978-3-030-82335-1_1
- Marconi A, Quadri M, Saltari A, Pincelli C. Progress in melanoma modelling in vitro. Experimental Dermatology. 2018;27(5):578-586. doi:https://doi.org/10.1111/exd.13670
- Niehues H, Bouwstra JA, El Ghalbzouri A, Brandner JM, Zeeuwen PLJM, van den Bogaard EH. 3D skin models for 3R research: The potential of 3D reconstructed skin models to study skin barrier function. Experimental Dermatology. 2018;27(5):501-511. doi:https://doi.org/10.1111/exd.13531
- Knight E, Przyborski S. Advances in 3D cell culture technologies enabling tissue-like structures to be created in vitro. Journal of Anatomy. 2015;227(6):746-756. doi:https://doi.org/10.1111/joa.12257
- Urciuolo F, Imparato G, Netti PA. In vitro strategies for mimicking dynamic cell-ECM reciprocity in 3D culture models. Frontiers in bioengineering and biotechnology. 2023;11:1197075. doi:10.3389/fbioe.2023.1197075
- Moniz T, Costa Lima SA, Reis S. Human skin models: From healthy to disease-mimetic systems; characteristics and applications. British Journal of Pharmacology. 2020;177(19):4314-4329. doi:https://doi.org/10.1111/bph.15184
- Mohammadi MH, Heidary Araghi B, Beydaghi V, et al. Skin diseases modeling using combined tissue engineering and microfluidic technologies. Advanced Healthcare Materials. 2016;5(19):2459-2480. doi:https://doi.org/10.1002/adhm.201600439
- Planz V, Lehr CM, Windbergs M. In vitro models for evaluating safety and efficacy of novel technologies for skin drug delivery. Journal of Controlled Release. 2016;242:89-104.
- Mathes SH, Ruffner H, Graf-Hausner U. The use of skin models in drug development. Advanced drug delivery reviews. 2014;69:81-102.
- Menon GK, Kligman AM. Barrier functions of human skin: a holistic view. Skin pharmacology and physiology. 2009;22(4):178-189.
- Bonaventure J, Domingues MJ, Larue L. Cellular and molecular mechanisms controlling the migration of melanocytes and melanoma cells. Pigment cell & melanoma research. 2013;26(3):316-325.
- Bertolini M, Piccini I, McElwee KJ. In vitro and ex vivo hair follicle models to explore therapeutic options for hair regeneration. Hair Follicle Regeneration. Published online 2022:155-203.
- Guerra A, Belinha J, Jorge RN. Modelling skin wound healing angiogenesis: A review. Journal of theoretical biology. 2018;459:1-17.
- Bergers LIJC, Reijnders CMA, van den Broek LJ, et al. Immune-competent human skin disease models. Drug discovery today. 2016;21(9):1479-1488.
- Ghose S, Ju Y, McDonough E, et al. 3D reconstruction of skin and spatial mapping of immune cell density, vascular distance and effects of sun exposure and aging. Communications Biology. 2023;6(1):718.
- Chouhan D, Dey N, Bhardwaj N, Mandal BB. Emerging and innovative approaches for wound healing and skin regeneration: Current status and advances. Biomaterials. 2019;216:119267.
- Filaire E, Nachat‐Kappes R, Laporte C, Harmand M, Simon M, Poinsot C. Alternative in vitro models used in the main safety tests of cosmetic products and new challenges. International Journal of Cosmetic Science. 2022;44(6):604-613.
- Zhang C, Zhang H, Ge J, et al. Landscape dynamic network biomarker analysis reveals the tipping point of transcriptome reprogramming to prevent skin photodamage. Journal of Molecular Cell Biology. 2021;13(11):822-833.
- Vidal Yucha SE, Tamamoto KA, Kaplan DL. The importance of the neuro‐immuno‐cutaneous system on human skin equivalent design. Cell proliferation. 2019;52(6):e12677.
- Beaumont KA, Mohana-Kumaran N, Haass NK. Modeling melanoma in vitro and in vivo. In: Healthcare. Vol 2. MDPI; 2013:27-46.
- Jin Z, Li Y, Yu K, et al. 3D printing of physical organ models: recent developments and challenges. Advanced Science. 2021;8(17):2101394.
- Vijayavenkataraman S, Lu WF, Fuh JYH. 3D bioprinting of skin: a state-of-the-art review on modelling, materials, and processes. Biofabrication. 2016;8(3):32001.
- Randall MJ, Jüngel A, Rimann M, Wuertz-Kozak K. Advances in the biofabrication of 3D skin in vitro: Healthy and pathological models. Frontiers in bioengineering and biotechnology. 2018;6:154.
- Zhang M, Zhang C, Li Z, Fu X, Huang S. Advances in 3D skin bioprinting for wound healing and disease modeling. Regenerative Biomaterials. 2023;10:rbac105.
- Stark HJ, Boehnke K, Mirancea N, et al. Epidermal homeostasis in long-term scaffold-enforced skin equivalents. In: Journal of Investigative Dermatology Symposium Proceedings. Vol 11. Elsevier; 2006:93-105.
- Sun H, Zhang YX, Li YM. Generation of skin organoids: Potential opportunities and challenges. Front Cell Dev Biol. 2021;9:3176.
- Sutterby E, Thurgood P, Baratchi S, Khoshmanesh K, Pirogova E. Microfluidic skin‐on‐a‐chip models: Toward biomimetic artificial skin. Small. 2020;16(39):2002515.
- Tung YC, Hsiao AY, Allen SG, Torisawa Y suke, Ho M, Takayama S. High-throughput 3D spheroid culture and drug testing using a 384 hanging drop array. Analyst. 2011;136(3):473-478.
- Souza GR, Molina JR, Raphael RM, et al. Three-dimensional tissue culture based on magnetic cell levitation. Nature Nanotech. Published online 2010:291-296.