
#1: 3D cell culture (continues) – a more and more developing field
Not a new trend for 2020 but a growing one nonetheless. In 2019 there were 72,000 papers published mentioning 3D culture compared with 46,000 in 2018. And, while the concept of 3D culture isn’t new, there are exciting developments and new approaches emerging.
Drug development is notorious for its high failure rate, with many a promising candidate failing to live up to its promise in clinical trials. This is a major source of financial losses for the industry.
The pharmaceutical industry hopes that better research in the early stages will help prevent expensive losses down the line, and human primary cell culture plays a key role in this.
Despite the many scientific advances that have cell culture to thank, 2D culture has its limitations in reflecting the normal behavior of cells seen in living tissue. 3D culture looks to overcome some of these because cells are not restricted to growing on one flat surface, but can stack on top and around each other, just as in tissue.
A common strategy is to culture the cells on a 3D scaffold. But what kind of scaffold and how is it engineered? Recent research has explored diverse approaches including graphene scaffolds (Vlăsceanu et al., 2019), nanofibers (Kim et al., 2019), 3D printing (Jian et al., 2018), freeze-casting (Jung et al. 2019), and even using natural marine collagen (Paradiso et al. 2019). Some studies have looked to lose the scaffold altogether using magnetic levitation to allow cells to assemble in 3D (Ferreira et al. 2019).
What’s certain is that this area of cell culture is growing rapidly and, in the process, it’s perfectly demonstrating the full creativity of scientific research.
#2: 4D cell culture – adding one further dimension to increase biological relevance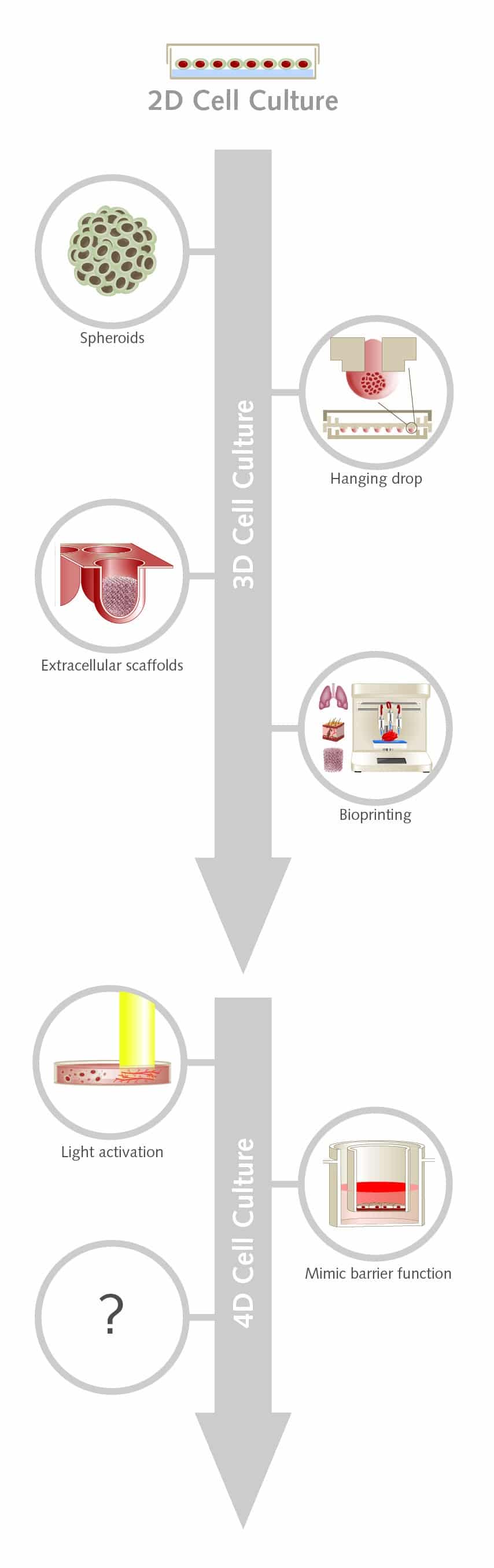
With interest in 3D cell culture growing, we could be already on the cusp of adding yet another dimension to cell culture. 4D culture takes the advantages of 3D cell culture but adds a culture medium that more closely mimics the extracellular matrix (ECM) of native tissue.
Most 3D systems are static so they can’t capture the dynamic nature of the ECM. The ECM interacts with cells and has a complex assortment of biochemical cues and physical properties that vary across space and time. Recreating this would advance the study of fundamental cell biology and could also enhance human tissue engineering.
Researchers have begun exploring ways that cell culture could be modified on demand during an experiment, without damaging the cells. One approach is to use light to alter the behavior of a photoresponsive hydrogel.
In a recent paper, (Farrukh et al., 2019) researchers generated a 4D cell culture, incorporating a light-activated peptidomimetic into their hydrogel. They cultured our human umbilical vein endothelial cells (HUVECs) in the presence of the growth factor VEGF and used a laser to activate the peptidomimetic.
Cells in light-exposed areas migrated extensively and formed a complex microvascular network in under a week. Unilluminated HUVECs did not show these changes and became non-viable within three days, despite the presence of VEGF.
The team said the findings show how the approach can control the culture environment in 3D space and time, allowing them to directly control angiogenesis with the simple use of light.
#3: Coming closer to biological reality
In the coming year, there will no doubt be continuing innovation in stem cell culture to help in vitro experiments more closely mimic biological reality.
In a recent study (Calejo et al., 2020), a team used a new approach to barrier substrate called the breath figure method. The technique was used to mimic the blood-retinal barrier in the eye, which is a critical location in the disease pathology of macular degeneration.
They cultured hiPSC-derived retinal pigment epithelium and endothelial cells on opposite sides of the barrier – a highly porous film with a honeycomb-like surface – which were both coated with collagen using a method known as the Langmuir-Schaefer technique.
Doing so enabled them to create a three-layered culture, where both layers of cells could freely exchange substances through the barrier, while maintaining physical separation.
The researchers note that the approach helps to avoid the use of in vivo animal models of the disease, while also addressing the limitations of existing in vitro models of the barrier which have been far too simple to reflect the function of this anatomy or the progression of the disease.
#4: Bioprinting
3D bioprinting has been embraced in the field of regenerative medicine because of its impressive potential in the construction of complex functional tissues and organoids (Jian et al., 2018).
The value of 3D bioprinting is no better demonstrated than in the bioprinting of blood vessels. Vasculature formation has been a longtime obstacle in tissue engineering, needing to be both precise and complex on an extremely small scale. Bioprinting now offers the tools to overcome these challenges.
Previous research has successfully used fugitive bioinks to create tubular matrices for endothelial cells to be seeded (Miri et al. 2018). The hydrogel is cast around the bioink which is subsequently flushed away, leaving behind a surface on which the cells can form a lumen.
In a recent study, (Tröndle et al., 2019) researchers looked to escape the need for fugitive bioinks or preformed channels by combining cellular self-assembly with 3D bioprinting using PromoCell HDMECs and HUVECs.
Using a drop-on-demand approach with endothelial cells in a suspended or spheroid bioink, they were able to form a lumen sandwiched between two layers of hydrogel. The bioprinting allowed them to dictate a number of biologically relevant structures and, after printing, cells showed self-assembly, including undirected branching into lumens with smaller diameters, as seen in the human vascular system.

#5: Simplifying research with HLA-typed cells
Human leukocyte antigen (HLA) molecules play a vital role in allowing the immune system to recognize antigens and mount a response against invading pathogens.
In research, this role is important in fields such as organ transplantation, where donor and recipient HLA types must be matched to prevent rejection, and in the development of cancer immunotherapy, an expanding and hugely promising field.
It can take up to several weeks to get results back from traditional HLA-typing, slowing down your research. To help streamline the process, we have a unique inventory of over 100 HLA-typed donor cells. These cells come from a range of human tissues, including the musculoskeletal system, kidney, bone marrow, peripheral blood cells, and more, which are ready to ship complete with their HLA-typing report.
A recent study (Aljabri et al., 2019) used HLA-typed cells to show that HLA type II antibodies can induce necrotic cell death in endothelial cells, through a complement-independent pathway. The endothelium is critical in the development of antibody-mediated rejection in solid organ transplantation so these findings could help inform strategies to prevent or treat rejection, the team say.
Looking into 2020
The steps we’ve all taken in 2019 make for an exciting year ahead with improvements and advances in the types, throughput and fundamental ideas in cell culture! We’re sure you are as excited as us to see what’s next. Check out our blog throughout the year for new releases.