What’s in vitro angiogenesis?
The formation of new blood vessels from pre-existing vasculature — a process known as angiogenesis — has been the subject of extensive research for years because of its importance in tissue development, repair, and disease progression.1 Unlike in vivo angiogenesis, which occurs within the complex environment of animal models and is influenced by numerous systemic factors, in vitro models offer the advantage of isolating specific variables and studying angiogenesis under controlled conditions.2
Why is in vitro angiogenesis important?
In vitro angiogenesis models have been instrumental in elucidating the steps involved in this process. These simplified systems enable the identification of cellular and molecular events that regulate angiogenesis, which would be challenging to achieve in a more complex in vivo environment.3
Moreover, in vitro angiogenesis assays allow for the systematic evaluation of the effects of various stimuli, such as growth factors, cytokines, and pharmaceutical compounds, on the different stages of blood vessel development.2 By isolating and culturing relevant cell types, such as endothelial cells, scientists can study the different steps of blood vessel formation and identify potential therapeutic targets for angiogenesis-related diseases.
Role of angiogenesis in physiological and pathophysiological processes
The formation of new blood vessels plays a pivotal role in various physiological processes, including embryonic development, tissue regeneration, and the growth and function of the female reproductive system.1 In adults, new blood vessels are formed to facilitate the delivery of oxygen and nutrients to the site of injury, promoting tissue repair and regeneration.1 During these processes, the formation of new blood vessels ensures the delivery of oxygen and nutrients to the growing or repairing tissues, supporting their growth and function.
However, the dysregulation of angiogenesis can also contribute to the progression of several pathological conditions, such as cancer, age-related macular degeneration, and diabetic retinopathy.4,5 In cancer, the formation of new blood vessels supports tumor growth and metastasis.6 Conversely, impaired angiogenesis in patients with cardiovascular conditions can contribute to the development of ischemic disease.7
Key events in angiogenesis
The formation of new blood vessels through angiogenesis occurs in multiple steps, orchestrated by a complex interplay of various growth factors, cytokines, and signaling molecules. Endothelial cells, which line the inner surface of blood vessels, play a pivotal role in all steps of this process.8
- Activation and proliferation of endothelial cells: Endothelial cells, the primary building blocks of blood vessels, respond to pro-angiogenic signals, such as VEGF, which activate their proliferation.9
- Degradation of the extracellular matrix (ECM): Activated endothelial cells secrete enzymes that degrade the basement membrane and surrounding ECM, creating a path for their migration and invasion.10
- Migration of endothelial cells: Activated endothelial cells migrate towards the site of new blood vessel formation, guided by a concentration gradient of angiogenic stimuli.11
- Formation of tube-like structures: As endothelial cells advance, they align themselves to form tube-like structures. These structures mature into stable blood vessels in response to various paracrine signals and the recruitment of supporting cells, such as pericytes.12
By modeling these events in a controlled laboratory setting, researchers can gain valuable insights into the complex interplay of cellular and molecular players involved. This paves the way for the development of targeted therapeutic interventions.
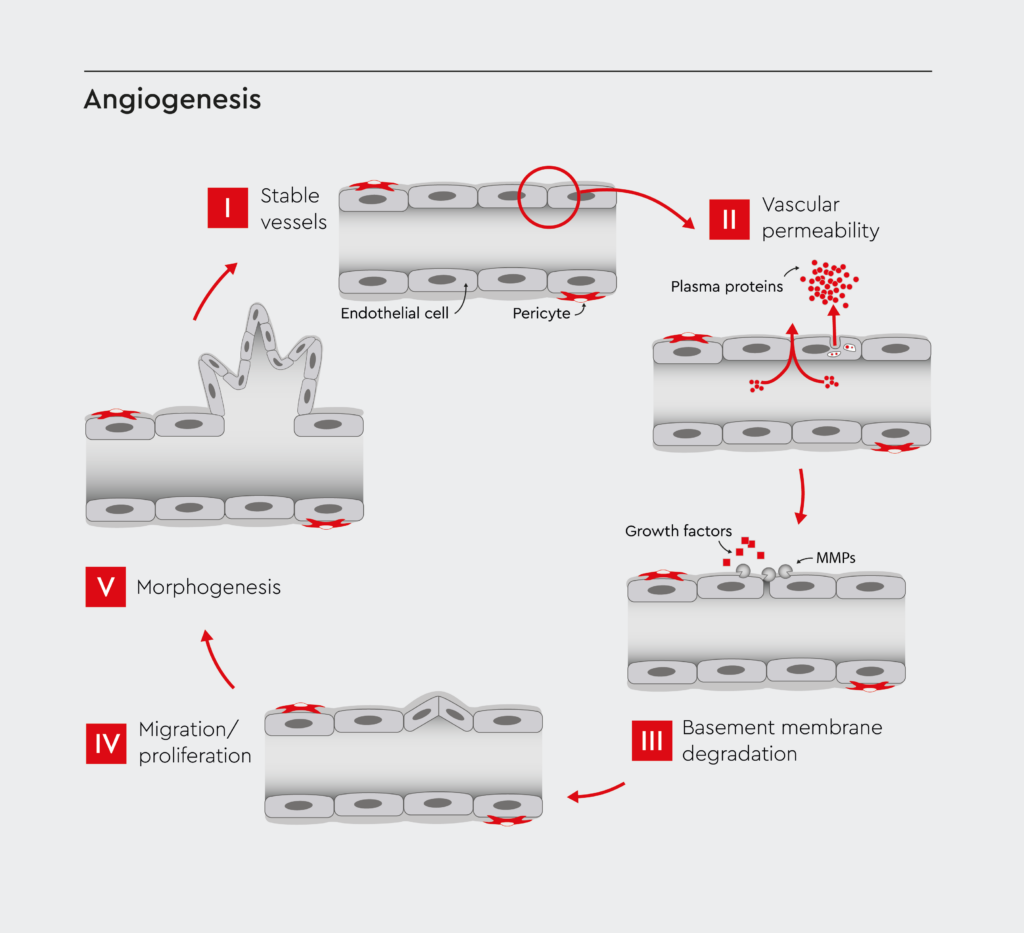
Factors regulating angiogenesis
Reflecting its important role in health and disease, angiogenesis is tightly controlled by various pro-angiogenic and anti-angiogenic factors and signaling pathways to ensure that it only occurs when and where needed.
- Vascular endothelial growth factor (VEGF) is considered the master regulator of angiogenesis, as it stimulates endothelial cell proliferation, migration, and tube formation.14
- Angiostatic (e.g., angiostatin) and angiogenic (e.g., angiogenin, angiopoietin-1, angiopoietin-2) factors regulate angiogenesis by influencing the growth, migration, and survival of endothelial cells and expression of other pro-angiogenic and anti-angiogenic genes.15
- Hypoxia, a hallmark of angiogenic microenvironments, further amplifies angiogenic signaling by activating hypoxia-inducible factors (HIFs) and promoting the expression of pro-angiogenic genes.16
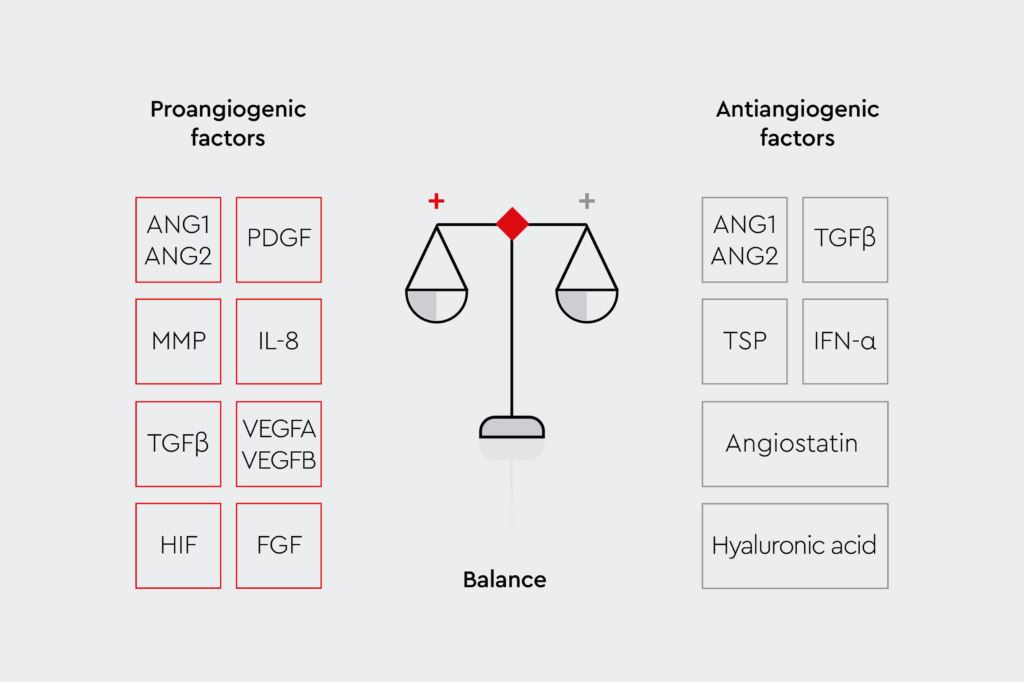
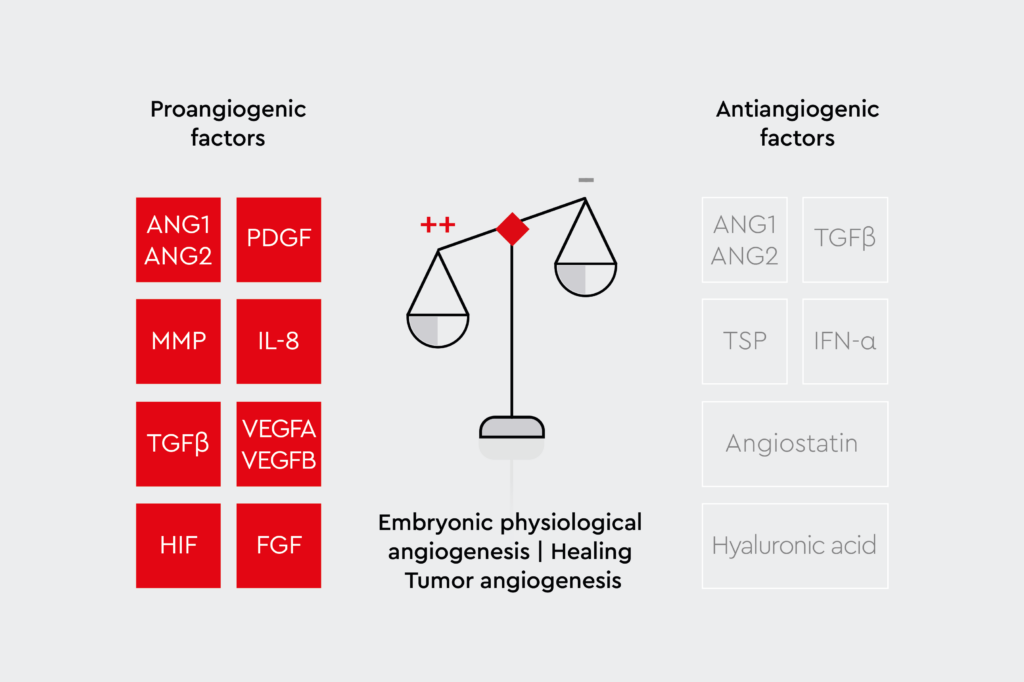
In vitro angiogenesis assays
In vitro angiogenesis models enable the investigation of key cellular players, signaling pathways, and environmental factors that influence blood vessel formation. Researchers can leverage various in vitro angiogenesis assays to study the effects of pro-angiogenic or anti-angiogenic agents on the different stages of blood vessel formation.
- Endothelial cell migration assays, such as scratch (undirected migration) or Boyden chamber assays (directed migration), can be used to evaluate the ability of endothelial cells to respond to angiogenic stimuli such as growth factors or chemotactic gradients by moving towards or away from the source of the signal.17
- Proliferation assays measure the rate of endothelial cell division, providing insights into the factors that promote or hinder their growth.18
- Tube formation assays capture the ability of endothelial cells to self-organize into intricate, capillary-like structures, providing insights into the morphogenic processes underlying angiogenesis.19
- Spheroid sprouting assays introduce a higher level of complexity by incorporating three-dimensional (3D) cell culture systems. This allows researchers to mimic the 3D environment of tissues and study cell-cell interactions and vessel branching in a more physiologically relevant context.20
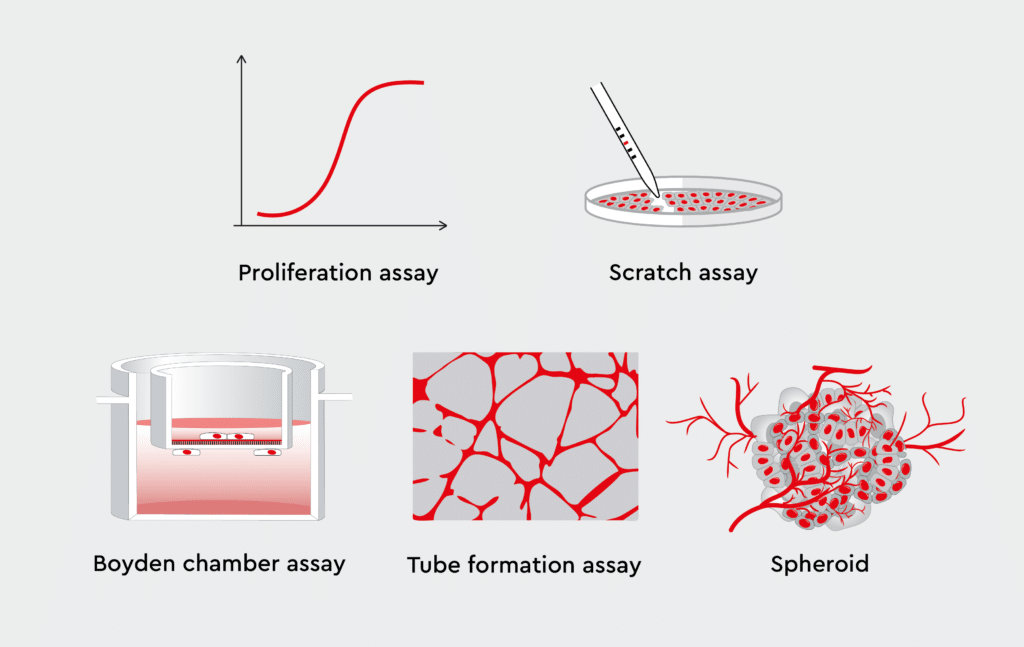
Although 2D assays provide a more controlled and high-throughput environment, 3D assays may better mimic the physiological conditions of the extracellular matrix and cell-cell interactions. The choice of assay depends on the specific research objectives, the desired level of complexity in the experimental setup, and the availability of relevant cell types and culture systems.17,18
Applications of in vitro angiogenesis assays
The versatility of in vitro angiogenesis models has led to their widespread application in various fields of biomedical research.
Drug discovery
In the drug discovery process, in vitro angiogenesis assays provide a valuable platform for rapid and cost-effective screening of candidate compounds. Researchers can assess the effects of these compounds on the different stages of the angiogenic process, identifying potential therapeutic agents that can either promote or inhibit blood vessel formation, depending on the targeted disease.21 This information can then guide the development of more targeted and effective therapies.
Disease modeling
In vitro angiogenesis models have been instrumental in establishing disease models for conditions such as cancer, cardiovascular diseases, diabetic retinopathy, and age-related macular degeneration.2,22 Researchers can study the underlying mechanisms of angiogenesis-related disease progression and evaluate the efficacy of novel therapeutic interventions by recapitulating the key features of these pathologies in a controlled laboratory setting.
Regenerative medicine
In vitro angiogenesis models have also been used to develop tissue engineering and regenerative medicine approaches.23 By understanding the factors that regulate this process, researchers can design biomaterials and scaffolds that support the formation of new blood vessels, facilitating the integration and survival of transplanted cells or engineered tissues.
Challenges and future directions for in vitro angiogenesis models
Despite their versatility, in vitro angiogenesis assays have limitations. Challenges in accurately recapitulating the complex in vivo environment and ensuring the relevance of in vitro findings to real-world scenarios are some of the challenges faced by researchers working with in vitro angiogenesis models.2 Factors such as the choice of cell types, the composition of the ECM, and the integration of supporting cell populations can significantly impact the outcomes of studies employing the formation of capillary-like structures in vitro.
Continuous efforts are being made to develop more sophisticated in vitro models that better mimic physiological and pathological vascular-related conditions observed in the human body. Future directions in in vitro angiogenesis research involve incorporating advanced technologies like:
- microfluidic systems,
- 3D tissue scaffolds,
- and organ-on-a-chip platforms,
to mimic physiological conditions more accurately and enhance the translational potential of these models.
Our solutions for in vitro angiogenesis research
As angiogenesis research continues to evolve, we remain at the forefront, offering high-quality tools and solutions to support your investigations. Our Human Umbilical Vein Endothelial Cells (HUVECs) and Human Dermal Microvascular Endothelial Cells (HDMECs) closely mimic the behavior and characteristics of endothelial cells found in the human body. These primary cells are ethically sourced from traceable, HLA-typed donors to ensure reproducibility.
The choice between the two cell types relies on the research question and cost.
- Macrovascular endothelial cells (e.g., HUVECs): Excellent and cost-effective in vitro model, able to capture the key features of angiogenesis.
- Microvascular endothelial cells (e.g., HDMECs): Physiologically relevant model, closely resembling the in vivo aspects of angiogenesis.
In addition to these cell types, we also offer a range of specialized cell culture media formulated to support the optimal growth and functionality of endothelial cells. We designed these media to maintain the angiogenic potential of cells, ensuring that you can recapitulate the complex processes involved in in vitro angiogenesis.
View recommended endothelial cell media guide
References
Expand
- Felmeden DC, Blann AD, Lip GYH. Angiogenesis: basic pathophysiology and implications for disease. Eur Heart J. 2003;24(7):586-603.
- Staton CA, Reed MWR, Brown NJ. A critical analysis of current in vitro and in vivo angiogenesis assays. Int J Exp Pathol. 2009;90(3):195-221.
- Vailhé B, Vittet D, Feige J-J. In vitro models of vasculogenesis and angiogenesis. Lab Investig. 2001;81(4):439-452.
- Ahmad A, Nawaz MI. Molecular mechanism of VEGF and its role in pathological angiogenesis. J Cell Biochem. 2022;123(12):1938-1965.
- Heloterä H, Kaarniranta K. A linkage between angiogenesis and inflammation in neovascular age-related macular degeneration. Cells. 2022;11(21):3453.
- Folkman J. Role of angiogenesis in tumor growth and metastasis. In: Seminars in Oncology. Vol 29. Elsevier; 2002:15-18.
- Griffioen AW, Molema G. Angiogenesis: potentials for pharmacologic intervention in the treatment of cancer, cardiovascular diseases, and chronic inflammation. Pharmacol Rev. 2000;52(2):237-268.
- Mariotti M, Maier JAM. Angiogenesis: an overview. New Front Angiogenes. 2006;1:1-29.
- Herbert SP, Stainier DYR. Molecular control of endothelial cell behaviour during blood vessel morphogenesis. Nat Rev Mol Cell Biol. 2011;12(9):551-564.
- Davis GE, Senger DR. Endothelial extracellular matrix: biosynthesis, remodeling, and functions during vascular morphogenesis and neovessel stabilization. Circ Res. 2005;97(11):1093-1107.
- Lamalice L, Le Boeuf F, Huot J. Endothelial cell migration during angiogenesis. Circ Res. 2007;100(6):782-794.
- Cleaver O, Krieg PA. Vascular development. In: Heart Development and Regeneration. Elsevier; 2010:487-528.
- Bryan BA, D’Amore PA. What tangled webs they weave: Rho-GTPase control of angiogenesis. Cell Mol Life Sci. 2007;64(16):2053-2065. doi:10.1007/s00018-007-7008-z
- Sousa Moreira I, Alexandrino Fernandes P, Joao Ramos M. Vascular endothelial growth factor (VEGF) inhibition-a critical review. Anti-cancer agents Med Chem (formerly Curr Med Chem agents). 2007;7(2):223-245.
- Distler JHW, Hirth A, Kurowska-Stolarska M, Gay RE. Angiogenic and angiostatic factors in the molecular control of angiogenesis. Q J Nucl Med Mol Imaging. 2003;47(3):149.
- Krock BL, Skuli N, Simon MC. Hypoxia-induced angiogenesis: good and evil. Genes Cancer. 2011;2(12):1117-1133.
- Stryker ZI, Rajabi M, Davis PJ, Mousa SA. Evaluation of angiogenesis assays. Biomedicines. 2019;7(2):37.
- Irvin MW, Zijlstra A, Wikswo JP, Pozzi A. Techniques and assays for the study of angiogenesis. Exp Biol Med. 2014;239(11):1476-1488.
- DeCicco-Skinner KL, Henry GH, Cataisson C, et al. Endothelial cell tube formation assay for the in vitro study of angiogenesis. J Vis Exp. 2014;(91):e51312. doi:10.3791/51312
- Shah S, Lee H, Park YH, et al. Three-dimensional angiogenesis assay system using co-culture spheroids formed by endothelial colony forming cells and mesenchymal stem cells. J Vis Exp. 2019;(151). doi:10.3791/60032
- Goodwin AM. In vitro assays of angiogenesis for assessment of angiogenic and anti-angiogenic agents. Microvasc Res. 2007;74(2-3):172-183. doi:10.1016/j.mvr.2007.05.006
- Malek G, Busik J, Grant MB, Choudhary M. Models of retinal diseases and their applicability in drug discovery. Expert Opin Drug Discov. 2018;13(4):359-377.
- Reddy LVK, Murugan D, Mullick M, Begum Moghal ET, Sen D. Recent approaches for angiogenesis in search of successful tissue engineering and regeneration. Curr Stem Cell Res Ther. 2020;15(2):111-134.
- Ahadian S, Civitarese R, Bannerman D, et al. Organ‐on‐a‐chip platforms: a convergence of advanced materials, cells, and microscale technologies. Adv Healthc Mater. 2018;7(2):1700506.
- Dellaquila A, Le Bao C, Letourneur D, Simon‐Yarza T. In vitro strategies to vascularize 3D physiologically relevant models. Adv Sci. 2021;8(19):2100798.
- Cochrane A, Albers HJ, Passier R, et al. Advanced in vitro models of vascular biology: human induced pluripotent stem cells and organ-on-chip technology. Adv Drug Deliv Rev. 2019;140:68-77.