3D models revolutionizing cardiovascular research
In the ever-evolving landscape of cardiovascular research, a groundbreaking frontier has emerged — the creation of bioprinted cardiac 3D models using primary human cells. Creating functional 3D models of the human heart has long been a holy grail for cardiovascular researchers. With recent advances in 3D bioprinting and tissue engineering, the ability to build a beating heart may soon be the reality.1 This cutting-edge technology promises to revolutionize the way we study and understand heart diseases, opening new doors to innovative treatments.
In this article, we will explore the pivotal role of cardiac models, the limitations of traditional 2D culture models, and the remarkable potential of novel 3D models for cardiovascular research. Moreover, we will delve into why adult primary human cells stand out as the most clinically relevant model for bioprinting cardiac spheroids and provide an overview of how our unique resources can help you bring a beating heart “to life” in your lab.
Breaking free from 2D constraints
For decades, cardiovascular research primarily relied on two-dimensional (2D) cultures of cardiac cells, but these models come with inherent limitations that hamper progress.2 Here are some of the key drawbacks:
- Low physiological relevance: 2D cultures lack the three-dimensional structure of the heart and fail to mimic the complex microarchitecture and multicellular environment of cardiac tissue.3 Therefore, it is challenging to study complex cardiac physiology using 2D cultures.
- Limited cellular crosstalk: Cells growing as monolayers in 2D cultures are often isolated. The limited cell-cell crosstalk in 2D cultures hinders the study of intricate cellular interactions among cardiac cells.4
- Incomplete modeling: 2D cardiac models poorly resemble the electrical and mechanical properties of real heart tissue.3 Therefore, traditional 2D models cannot capture the true complexity of diseases like heart failure or arrhythmias.
Cardiac spheroids: The heart of cutting-edge cardiovascular research
Cardiac spheroids have emerged as a promising 3D in vitro model of the human heart for the study of cardiac biology and disease. These self-assembled miniature 3D cardiac structures mimic the intricate architecture and function of the human heart while maintaining the convenience of an in vitro culture system.5
Cardiac spheroids hold immense promise and offer several key advantages over traditional 2D cardiac tissues:
- Physiological relevance: Cardiac spheroids closely emulate the human heart’s physiological conditions, making them an ideal model for disease modeling and drug testing.5
- True tissue architecture: 3D models replicate the layered structure of the heart, providing a more accurate representation of in vivo conditions.5,6
- Enhanced cellular communication: In 3D cultures, cells interact more naturally, fostering a deeper understanding of cardiac biology.5,6
- Spontaneous beating: Because cardiac cells in 3D cultures are electrically connected and interact more naturally, cardiac spheroids beat spontaneously with synchronized contractions.6,7
- Drug screening efficacy: Testing potential medications on 3D models yields more relevant and reliable results, speeding up the drug development process.7

Unveiling the full potential of cardiac spheroids
Innovative 3D cardiac models, such as bioprinted cardiac spheroids, open new avenues for improved diagnostics and treatment by bridging the gap between in vitro cell cultures and in vivo physiology of the human heart.
Composition of the human heart
The human heart is composed of various cell types, including cardiomyocytes, fibroblasts, and endothelial cells, which work together to maintain its structure and function.8 Cardiac myocytes are the force behind each heartbeat, whereas fibroblasts support cardiomyocyte function and survival. Endothelial cells promote tissue vascularization and nutrient supply.
3D culture systems, like cardiac spheroids, offer a closer approximation of the human heart than 2D cultures. However, even self-assembled cardiac spheroids have limitations. They contain only one or two cardiac cell types, and the cell organization is random rather than anatomically correct.9
This is where 3D bioprinting of primary human cardiac cells comes in.
Bioprinting cardiac spheroids using primary human cells
Bioprinted cardiac spheroids hold the potential to transform cardiovascular research by closely mimicking the human heart. By precisely positioning multiple cardiac cell types in pre-defined patterns, 3D bioprinting can recreate cardiac spheroids that contain the cell types and spatial orientation of the native heart.1,10 This approach relies on the use of adult primary human cells, such as cardiac myocytes, cardiac fibroblasts, and coronary artery endothelial cells.
With a mixture of cardiac myocytes, fibroblasts, and endothelial cells, bioprinted cardiac spheroids are currently the most physiologically relevant model, resembling the in vivo composition and structure of the human heart.1,5,10
Key advantages of bioprinted hearts include:
- Establishment of cardiac models composed of multiple cardiac cell types (cardiomyocytes, fibroblasts, endothelial cells)
- Vascularization for nutrient and gas exchange
- Electromechanical integration for synchronized beating
- Controlled architecture with anatomically correct geometry
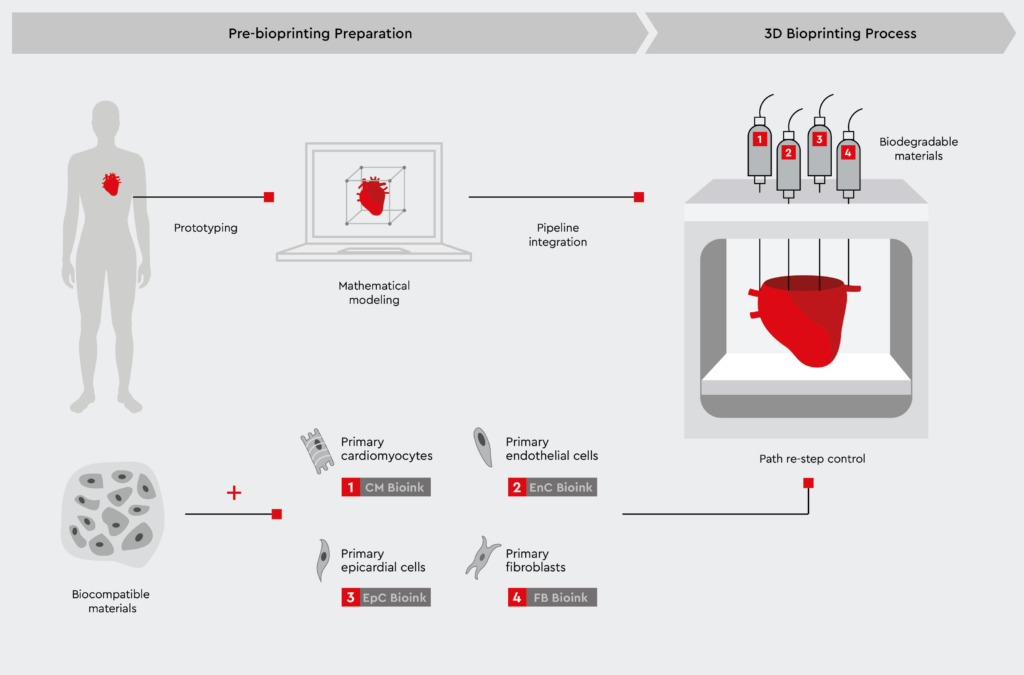
Cutting-edge applications of bioprinted cardiac spheroids
Bioprinted cardiac spheroids open new possibilities for personalized medicine. Cardiac spheroid bioprinting using primary cells from a biopsy can serve as a cutting-edge platform for the development and testing of personalized medicine. For example, bioprinted cardiac spheroids using cells from a biopsy can serve as patient-specific heart models to test innovative new drugs for heart diseases.11
3D bioprinting opens new avenues for regenerative medicine and can be used to generate advanced cardiovascular implants with biomimetic features.11 For example, this approach can be used to create patient-specific heart valves for the treatment of heart failure.12 Recent studies have also confirmed the feasibility of using 3D bioprinting to generate patient-derived cardiac tissue models to study congenital heart disease and personalized treatment strategies.13
Why primary human cells are key
To create clinically relevant cardiac 3D models, primary adult human cardiac cells are ideal.14 Here’s why:
- Better mimicry of adult heart physiology compared to stem cell-derived cells
- Patient-specific immunotype for personalized medicine
- Avoidance of genetic instability of commercially available cell lines
- Ability to isolate specific cardiac cell subpopulations
- Maturation state closer to native adult heart tissue
Innovative solutions for bioprinted cardiac 3D models
We provide a comprehensive portfolio of primary adult human cells, including all the necessary cell types for 3D cardiac models. Our primary human cells include:
- Primary Human Cardiac Myocytes (HCM): Our human cardiac myocytes are sourced from adult donors, ensuring reliable and reproducible results. These cells express cardiac-specific markers, such as sarcomeric alpha-actinin and slow-muscle myosin.
- Human Cardiac Fibroblasts (HCF): Complementing cardiac myocytes, our cardiac fibroblasts play a crucial role in maintaining the heart’s extracellular matrix and structure. They enable the creation of a more physiologically relevant microenvironment for your 3D cardiac models and allow for the study of cardiac fibrosis and remodeling, which are common features of heart diseases.
- Human Coronary Artery Endothelial Cells (HCAEC): To complete the tri-cell cardiac organ system, our coronary artery endothelial cells are essential. They facilitate vascularization, mimicking the blood vessel network within the heart.
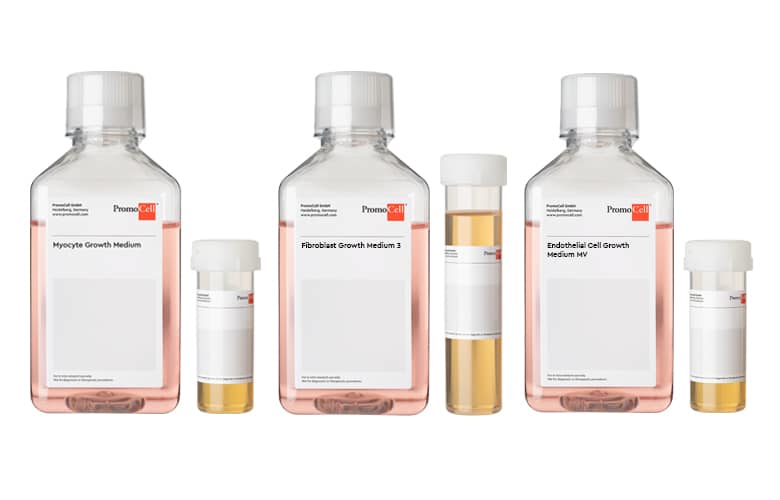
Creating bioprinted 3D hearts requires the use of clinically relevant and high-quality primary cells — that’s where our products shine. Our primary human cardiac myocytes, cardiac fibroblasts, and coronary artery endothelial cells are ideal for establishing bioprinted cardiac spheroids for several reasons:
- Authenticity: Our cells closely mirror the cells found in the human heart, ensuring the highest level of clinical relevance.
- Quality assurance: Rigorous quality control measures guarantee the purity and functionality of our cells.
- Consistency: We strive to serve as a consistent source of high-quality primary human cells for your experiments.
References
- Liu N, Ye X, Yao B, et al. Advances in 3D bioprinting technology for cardiac tissue engineering and regeneration. Bioact Mater. 2021;6(5):1388-1401. doi:10.1016/j.bioactmat.2020.10.021
- Pomeroy JE, Helfer A, Bursac N. Biomaterializing the promise of cardiac tissue engineering. Biotechnol Adv. 2020;42:107353. doi:10.1016/j.biotechadv.2019.02.009
- Abdelsayed G, Ali D, Malone A, et al. 2D and 3D in-Vitro models for mimicking cardiac physiology. Appl Eng Sci. 2022;12:100115. doi:https://doi.org/10.1016/j.apples.2022.100115
- Zhang P, Su J, Mende U. Cross talk between cardiac myocytes and fibroblasts: from multiscale investigative approaches to mechanisms and functional consequences. Am J Physiol Heart Circ Physiol. 2012;303(12):H1385-96. doi:10.1152/ajpheart.01167.2011
- Polonchuk L, Chabria M, Badi L, et al. Cardiac spheroids as promising in vitro models to study the human heart microenvironment. Sci Rep. 2017;7(1):7005. doi:10.1038/s41598-017-06385-8
- Polonchuk L, Surija L, Lee MH, et al. Towards engineering heart tissues from bioprinted cardiac spheroids. Biofabrication. 2021;13(4). doi:10.1088/1758-5090/ac14ca
- Zuppinger C. 3D culture for cardiac cells. Biochim Biophys Acta – Mol Cell Res. 2016;1863(7):1873-1881. doi:https://doi.org/10.1016/j.bbamcr.2015.11.036
- Souders CA, Bowers SLK, Baudino TA. Cardiac fibroblast: the renaissance cell. Circ Res. 2009;105(12):1164-1176. doi:10.1161/CIRCRESAHA.109.209809
- Thomas D, Choi S, Alamana C, Parker KK, Wu JC. Cellular and engineered organoids for cardiovascular models. Circ Res. 2022;130(12):1780-1802. doi:10.1161/CIRCRESAHA.122.320305
- Wang Z, Wang L, Li T, et al. 3D bioprinting in cardiac tissue engineering. Theranostics. 2021;11(16):7948-7969. doi:10.7150/thno.61621
- Cui H, Miao S, Esworthy T, et al. 3D bioprinting for cardiovascular regeneration and pharmacology. Adv Drug Deliv Rev. 2018;132:252-269. doi:10.1016/j.addr.2018.07.014
- Birla RK, Williams SK. 3D bioprinting and its potential impact on cardiac failure treatment: An industry perspective. APL Bioeng. 2020;4(1):10903. doi:10.1063/1.5128371
- Wolfe JT, He W, Kim M-S, et al. 3D-bioprinting of patient-derived cardiac tissue models for studying congenital heart disease. Front Cardiovasc Med. 2023;10:1162731. doi:10.3389/fcvm.2023.1162731
- Gisone I, Cecchettini A, Ceccherini E, Persiani E, Morales MA, Vozzi F. Cardiac tissue engineering: Multiple approaches and potential applications. Front Bioeng Biotechnol. 2022;10:980393. doi:10.3389/fbioe.2022.980393