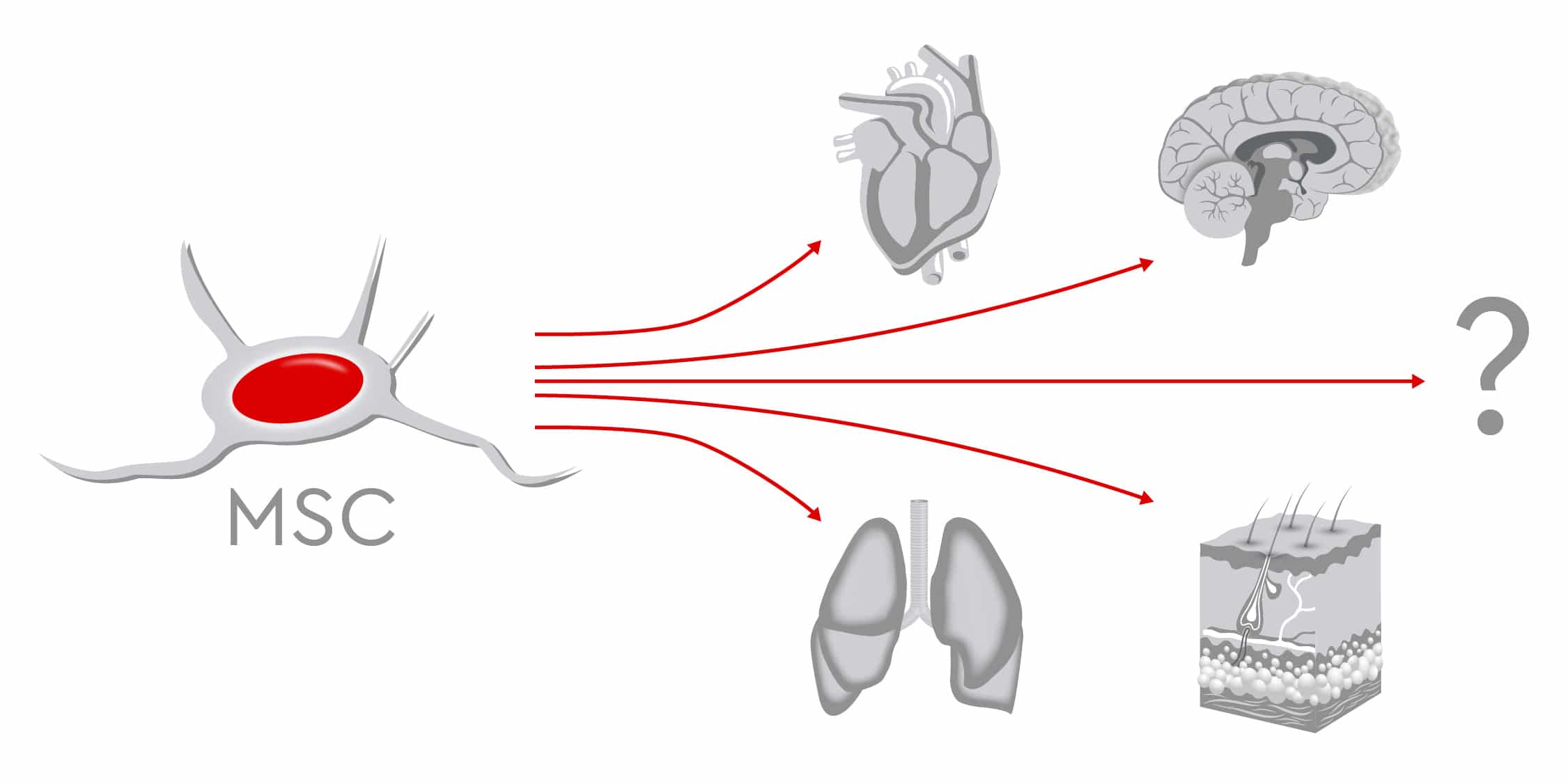
Imagine that millions of tiny maintenance workers inside the human body move around to injury sites to create new bones, cartilage, muscles and nerves. Within the crew is one particularly skilled craftsperson who has learned the secret of repairing almost all types of damaged tissues in the human body. Are mesenchymal stem cells (MSCs) this uniquely qualified master of repair? With more than 7,000 papers on MSCs published in 2018 and 788 complete or ongoing MSC-based clinical studies (Ayala-Cuellar et al., 2019), it is clear that MSCs have been the focus of intense research by both academia and industry since they were discovered by Friedenstein and colleagues in the 1970s. They possess unique self-renewing capacities and are able to differentiate into diverse lineages. As they are also easy to isolate and expand, MSCs are the most commonly used cells in regenerative medicine. In addition, because of their immunomodulatory properties, they offer promising treatment options for autoimmune, inflammatory, and hematological diseases, and in transplant surgery (Weiss and Dhalke, 2019).
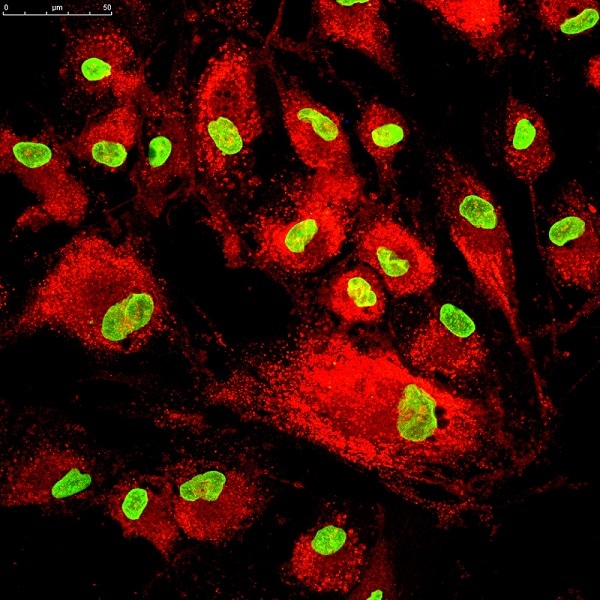
Expanding MSCs: from 2D monolayers to 3D bioreactors
Cell scientists harvest MSCs from sources including bone marrow, umbilical cord matrix, adipose tissue, tendon, lung, and the periosteum. They use autologous as well as allogeneic MSCs in clinical trials. As only low numbers of MSCs are present in the tissue of origin, they must be expanded in vitro to obtain enough cells for therapeutic purposes (Mizukami and Swiech, 2018). To expand MSCs, researchers generally rely on traditional monolayer cultures, which offer a simple, low-cost, and easy-to-use platform. For scaling up MSC expansion, roller bottles or multilayered cell factories can be used. However, new data suggest that 2D systems could limit the quality of MSCs, so using 3D systems could improve survival rates of MSCs, along with their stemness, anti-inflammatory, and angiogenic properties (Petrenko et al., 2017).
Cultivation in bioreactors produces high volumes of MSCs, ensures adherence to good manufacturing practices and guarantees high quality standards. Bioreactors continually monitor and regulate factors such as pH, temperature, oxygen, and carbon dioxide concentration. Once MSCs have been expanded, they must be collected and processed under optimal conditions to guarantee a highly viable and functional cell product. Quality control standards may vary between laboratories, as a consensus is missing on which exact parameters are needed. However, at least three criteria are needed to prove the MSC identity: adherence to plastic, expression of specific surface antigens and lack of others, as well as trilineage differentiation (Dominici et al., 2006). Other quality criteria include karyotype analysis, proof of high viability, and efficacy testing.
You are currently viewing a placeholder content from YouTube. To access the actual content, click the button below. Please note that doing so will share data with third-party providers.
Regenerative medicine moves from bench to bedside: MSC-based clinical trials
In preclinical and clinical trials, scientists continue to study the therapeutic potential of MSCs, to prove their safety and feasibility for various treatments. The findings show that MSCs successfully regenerate various tissues including bone, muscles, nerves, myocardium, liver, cornea, trachea, and skin (Han et al., 2019).
When bone defects occur after trauma, arthroplasty or tumor resection surgeries, physicians often recommend autologous or allogeneic bone grafting as therapy options. However, limited supply, risk of infections and complications during surgery restrict these procedures (García-Gareta et al., 2015 and Lozano-Calderón et al., 2016). Conversely, the osteogenic potential of MSCs makes them an optimal source for bone reconstruction. Numerous studies have compared the osteogenic ability of MSCs from different sources such as bone marrow, umbilical cord, and dental pulp, without reaching definitive conclusions as to which type is most suitable. Recent data indicate that human adipose-derived MSCs might have better proliferative capacities than bone marrow-derived MSCs, which means that MSCs from lipoaspirates could provide good candidates for clinical bone tissue engineering (Burrow et al., 2017).
In bone joints, cartilage does not repair itself well nor quickly due to the lack of blood supply. Current cartilage-repair techniques, such as bone marrow stimulation and osteochondral transplantation, show limited success. This has prompted scientists to search for valid alternatives and test new approaches. Beginning with preclinical trials in the 1990s, MSCs have been used for cartilage regeneration. Novel techniques use 3D scaffolds, which resemble the extracellular matrix, to optimize MSC proliferation and differentiation. Hydrogels loaded with MSCs and stimulating factors such as BMP-2/-4, insulin-like growth factor-1, and TGF greatly encourage repair of cartilage damage (Yang et al., 2017). Despite the high number of clinical trials, scientists do not yet agree on the optimal cell source, and additional studies are needed before MSCs can be routinely used to promote cartilage regeneration.

In addition to bone and cartilage, MSCs are also considered good candidates for supporting the regeneration of other musculoskeletal tissues such as ligaments, tendons and intervertebral discs. As the population ages, more patients acquire degenerative diseases of the spine, suffer severe back pain and require surgery. MSC-based therapies could represent valid alternatives to surgery for repairing intervertebral discs, preserving biomechanics, and achieving pain relief (Orozco et al., 2011).
Further characteristics of MSCs qualify them to regenerate nervous tissues as well. They can differentiate into neuron-like cells in vitro and in vivo and express neuronal markers. Regenerative efforts focus mainly on two areas: damage caused by severe trauma or ischemia, and dysfunction caused by neurological diseases such as multiple sclerosis, amyotrophic lateral sclerosis, ischemic stroke, and Parkinson’s disease. Through the release of several cytokines and other bioactive molecules such as TGF-ß, which regulate damage and repair processes, MSCs regulate the immune response and protect neuronal structures (van Velthoven et al., 2012). This means MSCs may support positive outcomes following brain injuries and when repairing peripheral nerves. Preclinical studies have confirmed that MSCs can increase recovery of function; however, only a few reports on clinical applications exist (Papa et al., 2018).
Going a step further, clinical trials and animal models have demonstrated that MSCs can regenerate not only tissues but entire organs such as the heart, liver, cornea, and trachea. Transplanted MSCs can stimulate the resident cardiac stem cells, differentiate into cardiomyocyte-like cells, or rebuild the blood vessels after myocardial infarction (Miao et al., 2017). In patients with cirrhosis, MSCs also contribute to improved liver function. In fact, MSCs can differentiate into several types of liver cells and can secrete trophic and immunomodulatory factors that support hepatocyte function, reverse fibrosis and promote angiogenesis (Wang et al., 2017). For researchers, developing this approach for treating liver failure is urgent, considering the high numbers of patients waiting on transplantation lists, compared to the relatively low number of available organs.
Other examples of MSCs used in regenerative medicine include the treatment of kidney injuries and lung diseases as well as regeneration of large-scale damage to the skin (Han et al., 2019).

Future challenges
As the fields of MSC research is growing exponentially, gaps in our basic knowledge of MSCs still limit our full understanding of their biological functions. In a recent comment in Nature, Sipp et al., prompt the scientific community to “clear up this stem-cell mess.” The authors state that MSCs have acquired a “near-magical, all-things-to-all-people quality in the media and in the public mind,” becoming the “go-to cell type for many unproven stem-cell interventions.” They point out that even if MSCs increase recovery of function in preclinical studies, the majority of clinical studies are still in the early stages. Only a few commercial MSC-based products have been approved by regulatory agencies so far.
For clinical applications, the safety of MSC therapies must be guaranteed. The systemic infusion of MSCs appears relatively safe, but there is a risk that such infusions also induce tumors, inflammatory responses, and fibrosis (Fitzsimmons et al., 2018). In some instances, MSCs could transform into malignant cells or promote tumor formation. As MSCs suppress the immune system and encourage formation of new blood vessels, these properties can also support tumor growth and metastasis. During repair processes, fibrotic reactions could also take place, as MSCs can also differentiate into fibroblasts.
In addition, researchers still must overcome obstacles to large-scale production of MSCs. Because MSC populations are heterogeneous, they require different platforms and processes for different indications. Similarly, because every application requires a tailored, specific protocol, producing these cellular products involves high costs. The lack of consensus on quality control standards also hinders the standardization of cell manufacturing bioprocesses. Although bioreactors allow the expansion of cells in a controlled environment, assessing product safety and efficacy remains challenging. MSCs cannot be kept in culture for too long, as they might then lose important functions, so scientists must carry out expansion of cell populations promptly. In addition, serum-free, chemically defined media should be used to avoid ethical issues and immunological reactions related to the use of animal sera. Researchers must ensure complete absence of contamination, as cellular products cannot undergo viral inactivation processes similar to those used for recombinant proteins.
MSCs are a multifaceted cell population offering a range of functions and a remarkable therapeutic potential. Yet, before the “maintenance crew” can be sent to repair damaged tissue on a large scale, scientists need better understanding the tools that the workers have at their disposal.